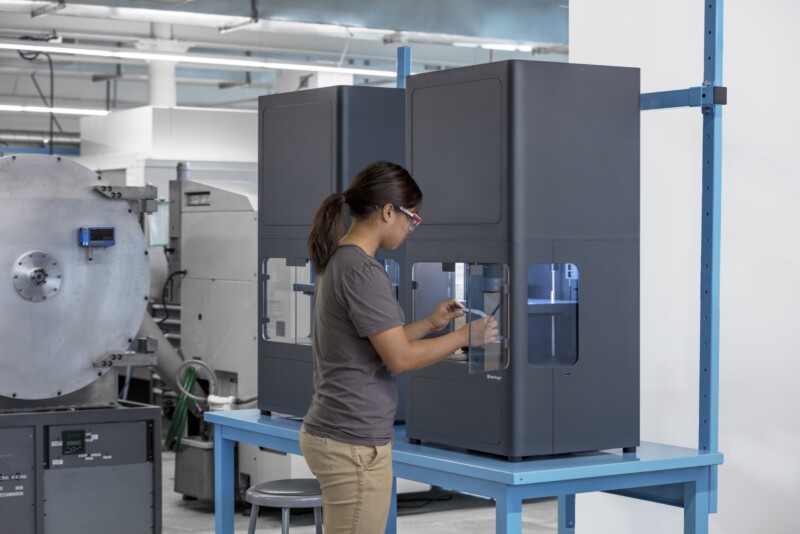
What is Additive Manufacturing? Understanding the Technology and its Impact
What is additive manufacturing?
The term additive manufacturing (AM) refers to the process of fabricating physical 3D objects by extruding material, layer-by-layer, to form the desired shapes. For manufacturers, AM typically refers to industrial use of 3D printing for activities such as building tooling and fixtures, prototyping and design validation, and production of low-volume end-use parts.
Why is it called additive manufacturing?
The etymology of the term “additive manufacturing” comes from the methodical contrast between how 3D printing and traditional “subtractive” manufacturing processes work to shape objects. 3D printers work through the addition of layers of material in different shapes on top of each other. Traditional manufacturing methods produce the final result through the reduction or subtraction of material, such as cutting away sections of an alloy to make a bolt or hinge. Traditional subtractive manufacturing is notoriously slow, expensive, and comes with design limitations, whereas additive manufacturing is paving the way for quick, low-cost, automated processes.
Additive manufacturing and 3D printing
Within the industry, additive manufacturing will typically always refer to 3D printing. Technically speaking however, 3D printing is a subset of AM, which includes a few other non-3D printing methods of manufacturing.
While the terms “3D printing” and “additive manufacturing” are frequently used interchangeably, one key distinction should be noted. Additive manufacturing typically carries an industrial connotation, often referring to larger-scale use of 3D printing for manufacturing operations.
This distinction is important to note as the industry includes many cheaper, smaller-scale 3D printers. These do not satisfy the same purpose: they are typically employed as gimmick factories for novelty items, and are not designed to perform in actual high-value manufacturing applications.
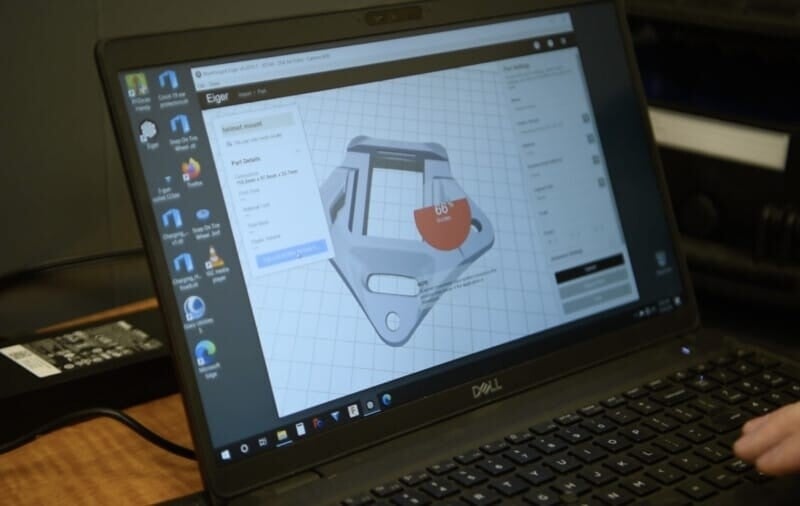
How does additive manufacturing work?
Additive manufacturing works by converting digital inputs — such as computer-aided design files of industrial parts — into tangible 3D objects.
Software. Once a user has a digital CAD file for their part(s), they will export the design as a .STL (Standard Tessellation Language) file. STL is the global industry standard file type for 3D printing. An STL is a solid-body representation of a part that 3D printing software can parse and turn into printing instructions.
The user then imports the part design as an STL file into an additive manufacturing “slicer” software. The slicer software — according to the user’s various part and print settings — then translates the STL file into a set of machine instructions for the 3D printer.
With APIs, 3D printing software can integrate with factory systems, such as ERP or MES systems, to streamline and automate operations.
Hardware. The 3D printer then uses the machine instructions to determine the patterns in which it extrudes filament material. The printhead is programmed to move across both horizontal (X-Y) and vertical (Z) axes, and deposit material across points across the XY and Z axes according to the 3D printing software’s directions. The 3D printing process builds objects from the bottom up — each additional horizontal layer is then stacked atop the previous layer. Print jobs are finished after completion of the uppermost layer.
Materials. For plastic and composite 3D printers, additive manufacturing typically uses spools of filament. 3D printers heat the filament into a molten plastic that it can extrude through a very small nozzle for precise placement. After it completes each layer, the material dries and hardens, after which the next layer can be printed.
Additive manufacturing with metals works differently than 3D printing plastics. These 3D printing materials are typically presented as a metal powder. This is necessary because of the high melting temperatures for metals. A 3D printer’s extrusion system cannot survive prolonged contact with molten metal, making extrusion of metals not feasible. Therefore to additively manufacture metals, parts must first be printed in powder form and then converted into a uniform, fully metal part through a high energy process — such as lasering, or sintering in a furnace.
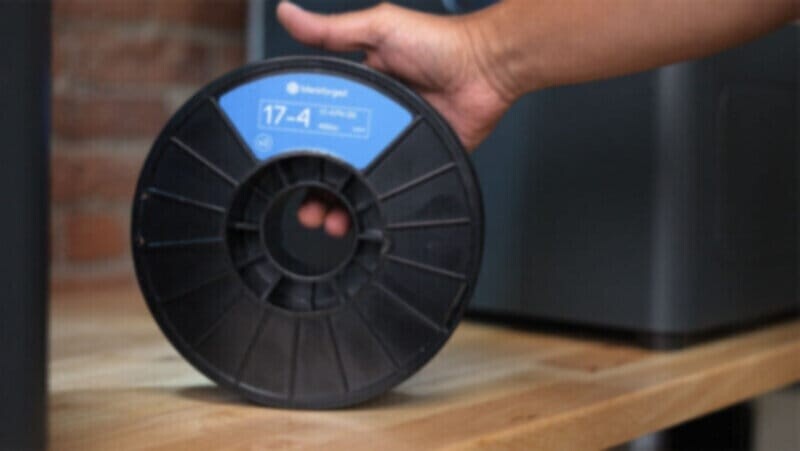
Additive manufacturing materials
Plastics. Widely used plastics range from budget-friendly prototyping materials like to rubbery, flexible filaments and high-performance thermoplastics like ULTEM™ 9085 Filament. Plastic materials are typically packaged in filament spools. Widely used plastics in 3D printing include:
- Nylon is a flexible, durable plastic material with good resistance to impact and chemical exposure.
- PLA (Polylactic Acid) is a cost-effective thermoplastic often used for rapid prototyping.
- TPU (Thermoplastic Polyurethane) is a flexible, impact-resistant rubber-like material.
- ABS (Acrylonitrile Butadiene Styrene) is another economical material. While a little weaker than PLA, it is lighter and more durable.
- ULTEM™ 9085 Filament is a high-performance thermoplastic used for demanding applications that require very high durability, temperature resistance, and chemical resistivity.
Metals. AM metals typically come in powder form, either as loose powders or bound together with a binder material. Popular available materials include:
- 17-4PH stainless steel is a versatile, common metal used for a variety of industrial manufacturing applications.
- A2 and D2 tool steels are cold work tool steels that offer extremely high hardness after heat treatment.
- H13 tool steel is a hot work tool steel that maintains material properties at high temperatures.
- Copper is often used for thermal and electrical operations. It conducts heat and electricity better than traditional metals.
- Inconel is a superalloy based on nickel and chromium. Inconel is used for applications that require resistance to corrosion, high temperatures, and chemicals.
Composites. Some FDM-printers can create composite materials combining plastics with reinforcing fibers to improve the parts’ strength, durability, stiffness, and heat resistance. Widely used fiber materials combined with plastics to form composites include:
- Carbon fiber is ideal for high-strength applications, with a 50% better strength-to-weight ratio than 6061 aluminum, a tensile modulus roughly equivalent to aluminum, and a stiffness 24 times higher than ABS. Flame retardant carbon fiber varieties are often used in aerospace, automative, and transportation industries.
- Kevlar® Aramid Fiber is ideal for applications that require extreme durability, shock resistance, and impact resistance.
- Fiberglass is a cost-friendly all-purpose fiber that is three times stronger and 11 times stiffer than ABS.
- HSHT (High strength, high temperature) fiberglass maintains its properties at extremely high temperatures compared to other fibers — even up to 200°C.
For more information about continuous fibers and their role in 3D printing composites, read this article.
*The ULTEM™ and 9085 trademarks are used under license from SABIC, its affiliates or subsidiaries.
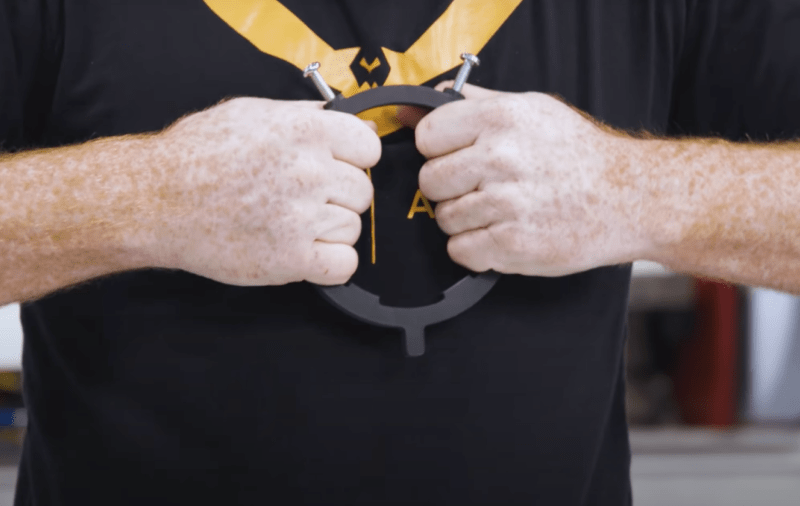
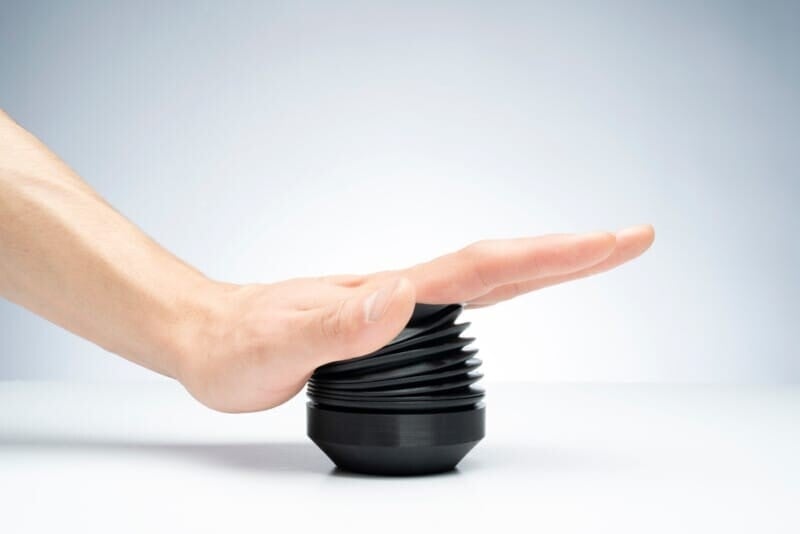
Benefits of additive manufacturing
Compared to other traditionally used methods of fabrication, additive manufacturing offers distinct technical and business benefits:
More parts can be produced in-house. When companies outsource a core manufacturing competency to a third party, they establish a dependency for tooling, fixtures, and jigs needed to produce the final product. As a result, manufacturers forfeit control and are subject to higher costs, prolonged timelines, and decreased transparency; while quality issues and other complications require additional time and coordination to address. Producing parts in-house also allows companies to better protect intellectual property, proprietary innovations, and other trade secrets.
Cost efficiency. Additive manufacturing is typically far more cost-effective than traditional subtractive manufacturing. 3D printing tooling for production can save manufacturers tens of thousands of dollars per month compared to machining. For most manufacturers, additive manufacturing platforms will yield a near-immediate ROI: within months, or even weeks.
Design freedom and process flexibility. The processes used to manufacture parts are oftentimes dictated by the limitations of what the traditional manufacturing process supports. For example, when manufacturing a bracket using subtractive techniques, unnecessary constraints are imposed by having to start with sheet metal, while bending or stamping into shape.
With additive manufacturing, designs can be made without these steps and limitations. Furthermore, 3D printing can produce complex geometries that are not achievable through other manufacturing techniques.
Faster lead times. The time it takes additive manufacturing to go from art to part is just a fraction of what it takes through subtractive manufacturing processes. The cycle from when the need for a part is recognized — all the way to when it is implemented — can be cut down to just hours or days, compared to months, as long as 3D printers are available.
Increased speed to market. Access to in-house additive manufacturing accelerates design cycles by enabling rapid prototyping. It can produce any part in a fraction of the time it would take to request and receive via traditional manufacturing processes.
Full supply chain control. Use of a 3D printing platform allows businesses to control their full supply chains from end to end. Manufacturers can reduce dependence on external suppliers and minimize risk in their supply chain operations.
Building a culture of innovation. For job-seeking engineers, companies invested in an additive manufacturing strategy present opportunities to focus on innovation and solve interesting design problems, while automating the arduous tasks and eliminating unnecessary constraints that come with subtractive manufacturing processes.
Engineers with access to in-house 3D printing do not have to worry about time-consuming procurement activities like drafting drawings, submitting purchase orders, and managing the bidding process with multiple vendors.
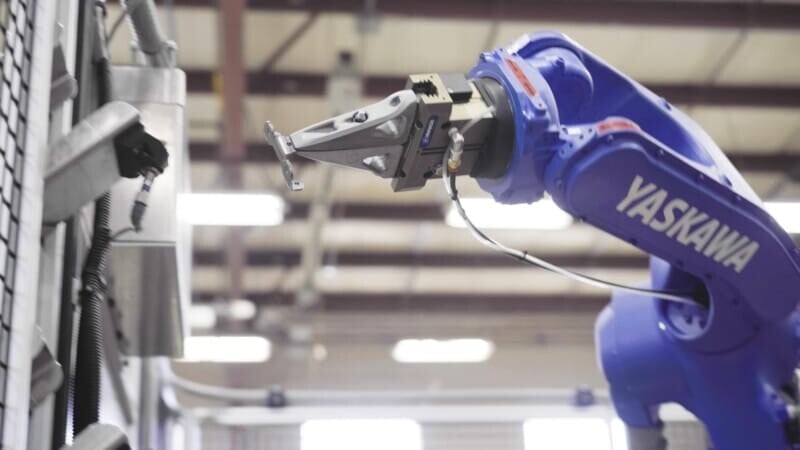
Potential drawbacks of additive manufacturing
Additive manufacturing carries many key benefits for numerous types of businesses. However, its use may be disadvantageous for specific scenarios and use cases.
Mass production costs. While the unit economics of printing parts is favorable in low volume production runs, AM is more expensive per unit when building parts in large quantities.
Limited throughputs. Historically, adoption of additive manufacturing has been hindered by speed and build size limitations. However, vendors have introduced several newer 3D printers to address these needs.
Software integration restrictions. Generally, the industry’s software platforms are vendor-specific and do not integrate well with factories and across inter-vendor machines. Only select providers offer reliable API integrations across factory systems.
Material costs. Materials may cost more in 3D printable formats compared to the same material in other forms. This is due to significant processing needed to render materials into an AM-friendly form.
State of current additive manufacturing technology
While additive manufacturing technologies have been commercially available since back in the 1980s, the industry and technologies have evolved significantly. Earlier 3D printers lacked the capabilities to make end use-quality parts, which limited widespread use to rapid prototyping applications.
Today’s additive manufacturing platforms distinguish themselves from earlier 3D printers in three main ways:
Hardware improvements. Today’s printers feature significantly improved speed, power, reliability, part strength, and print quality
Connectivity to Industry 4.0. Modern additive manufacturing platforms Industry 4.0’s suite of digital technologies — such as cloud computing, data analytics, Internet of Things (IoT), automation tools, and software integrations — and apply them to the process of fabrication itself.
Broader material compatibility. Additive manufacturing platforms now incorporate a wider selection of materials, including various high-performance composites and metals. The new materials open up applications in new industries.
Standards. ISO/IEC 27001 certification on select additive manufacturing platforms, adhering to rigorous data security, privacy, confidentiality, integrity, and governance standards.
How organizations use AM across industries
Today, leading organizations across a wide range of industries incorporate additive manufacturing to address specific manufacturing needs:
Aerospace. Major aircraft OEMs use additive manufacturing in their operations. AM allows them to streamline their supply chains by printing strong, lightweight end-use parts for aircraft, as well as print tooling quickly and economically.
Consumer products. Additive manufacturing is used increasingly for end-use production parts on products such as audio equipment and electronic devices.
Dental. Dentists and orthodontists use additive manufacturing to fabricate dental models, dentures, retainers, aligners, dentures, and more.
Education. Leading universities are implementing additive manufacturing — in labs, makerspaces, and as part of engineering curricula — to educate the next generation of scientists, engineers, and manufacturers.
Energy. Leading energy providers 3D print parts that make the manufacture and maintenance of wind turbines faster, easier, and more efficient than ever.
Federal and Defense. Federal government organizations, such as the U.S. Air Force and Army, use additive manufacturing to accelerate R&D and solve supply chain issues with the ability to print mission-critical end-use parts from remote locations.
Industrial Equipment. Industrial manufacturers use additive manufacturing to build custom tooling, accelerate go-to-market timelines, and print end-use parts for various types of factory machine systems.
Medical. To meet production needs through strained supply chains, manufacturers of medical devices and equipment are 3D printing everything from tourniquet clips to COVID-19 personal protective equipment.
Scientific and Laboratory. Scientific manufacturers 3D print end-use parts for various laboratory automation systems.
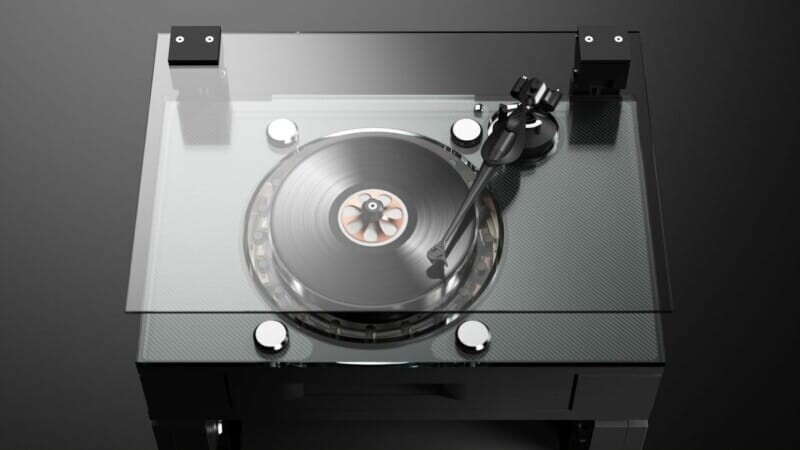
Types of additive manufacturing processes and technologies
All additive manufacturing technologies achieve the same purpose of building 3D objects from CAD designs by extruding material layer-by-layer. However, there are multiple ways of doing this. Different additive manufacturing processes offer different benefits and drawbacks
Fused Deposition Modeling (FDM®), or Fused Filament Fabrication (FFF), is a common additive manufacturing process that extrudes thermoplastic filament layer-by-layer on a build plate to form the object.
Advantages include cost effectiveness as well as parts with high strength, low weight, dimensional stability, and heat tolerance. Drawbacks include potentially anisotropic parts, which means strength across different directions may be uneven.
Binder Jetting fabricates parts out of powder media, which is spread along the print bed layer-by-layer to create the 3D object. After each layer of material is printed, a liquid binding agent is used to adhere the powder into a solid shape before the next layer is printed.
Benefits of binder jetting include its speed, precision, and cost effectiveness, as binder jetting machines use multiple heads to simultaneously jet binding material at many different spaces during a print. Tens, or even hundreds of parts can be printed in each build. Disadvantages include less part strength compared to other metal additive manufacturing processes.
Stereolithography (SLA) works by curing layers of a photopolymer (a light-sensitive liquid plastic) to create a part or object. SLA exposes each layer to an ultraviolet (UV) laser. This immediately hardens, or ‘cures’ each layer; each additional layer is cured and bonded to the previous layer until the object is complete.
Additive manufacturing through SLA is ideal for printing accuracy, detail, intricacy, and cost of equipment. However, larger parts are at risk of warping, and objects produced through this process are not particularly strong.
Selective Laser Sintering (SLS) is another additive manufacturing process. SLS uses lasers to ‘sinter,’ or bind together material — which comes as a fine powder — into the final object. The 3D printer creates the specific shape by aiming its laser at specific points of the powder bed.
Sintering causes an atomic reaction that fuses the particles of material powder into the solid object.
Benefits of SLS include its precision and isotropic (equal strength in every direction) strength. Downsides of using SLS for additive manufacturing include the high costs of machines, maintenance, and the skilled operators that are required to operate SLS printers.
Direct Metal Laser Sintering (DMLS) is an additive manufacturing technique that is also referred to as Selective Laser Melting (SLM). Similar to SLS, each layer is formed by aiming a laser at the powder bed in specific points within the space. After each layer is completed, the printer spreads powder for the next layer and repeats the process. Unlike SLS however, DMLS fully melts particles together.
Advantages for DMLS include stable mechanical and material properties, wide material availability, and repeatability of results after a successful print. Disadvantages of using additive manufacturing with DMLS include difficulty of purchasing and operating equipment. DMLS printers require skilled operators, additional supporting machinery (such as EDM machines), and careful management of powder material. Furthermore, printing accurate and functional parts typically requires a trial and error process.
Metal Fused Filament Fabrication (Metal FFF) starts with a plastic-bound metal powder made into the shape of a part, one layer at a time. The part is then washed in a debinding solution and sintered in a furnace — to burn off the plastic binder and cause the metal powder to diffuse together.
Benefits to using ADAM include wide material availability, cost effectiveness, accuracy, and use of bound powder. Compared to other metal additive manufacturing processes, which make use of loose powder, bound powder is safer and easier to use. A potential disadvantage (but also a potential advantage) of this additive manufacturing process is that the fabricated parts are not fully solid, instead with a triangular infill. While the resultant parts do not have full density, they have considerably lower weight.
Digital light processing (DLP) works similarly to SLA. The one key difference of this process is that instead of curing each layer of media with a laser tracing through each layer, DLP printers use a projected light source to cure the entire layer at once.
Benefits of DLP include its speed and capacity for accurately producing intricate designs. Limitations include a strong odor during the printing process, while large parts are at risk of warping.
Directed Energy Deposition (DED) fabricates parts with metal feedstock (consisting of either metal wire of powdered metal) and a laser. This process uses a nozzle mounted on a multi-axis robotic arm. As a result, material can be deposited at virtually any angle. Then, once deposited, the metal feedstock is melted with a laser or electron beam.
Benefits of DED include its ability to create larger metal parts than other processes, and its ability to add material to repair existing parts. Drawbacks include high equipment costs, the need for skilled technicians, a dedicated room for handling powder media, and necessary post-processing to improve accuracy.
Electron Beam Melting (EBM) melts powdered metal layer-by-layer with a high-energy beam of electrons, which are guided by a magnetic field. Fabrication takes place in a vacuum chamber.
Benefits of EBM include speed, accuracy, as well as strength of parts it produces. Disadvantages include requiring a skilled technician to operate each printer.
Multijet Printing (MJP), or Material Jetting (MJ), is an additive manufacturing process that deposits droplets of photoreactive material in the shape of each layer. After each layer, the printers apply UV (ultraviolet) to solidify the material.
Advantages include the ability to print parts consisting of multiple materials and/or colors, as different printheads can dispense different materials within a single layer. Disadvantages include strength and durability of printed parts.
Additive manufacturing and supply chains
Supply chain shortages pose critical threat to manufacturing operations today. Recent supply chain shortages are taking place on a global scale, and companies have been experiencing unprecedented levels of difficulty procuring the parts they need. Without the ability to quickly fabricate the needed parts in-house, manufacturing operations can easily stall for however long it takes for the part to be built, shipped, transported, and received. This often takes months.
Access to on-site additive manufacturing allows companies to take full control of their supply chains. Individual printers can be placed across different geographic locations; parts stored in a cloud-based digital inventory can then be sent to be printed at any 3D printer within the network. The right parts can be quickly printed at the precise locations where they are needed, when they are needed, with far shorter lead times compared to traditional manufacturing.
For parts that must be machined, using an additive manufacturing system for rapid prototyping will help engineers ensure that when the machined part is finally received months later, it will work as expected.
Read this article to learn how companies use additive additive manufacturing to reinvent their supply chains with drastically reduced lead times, better unit economics, and manufacturing at the point of need.
Additive manufacturing in Industry 4.0
Today, additive manufacturing is a significantly more effective manufacturing technology than it was even just 5-7 years ago. There are two reasons for this, alongside vastly improved speed, power, and reliability of 3D printing machines, the development of Industry 4.0 technologies has changed the way humans interact with these machines.
In simple terms, Industry 4.0 describes the broader digitization of the manufacturing industry. The term encapsulates industrial uses of technologies like data and analytics, Artificial Intelligence (AI), cloud computing, the Internet of Things (IoT), AR/VR, and automation — and their cohesive roles in the “smart factory” concept.
Additive manufacturing is a technology native to Industry 4.0, digitizing part design and production and automating fabrication. But the application of other technologies in the Industry 4.0 suite into AM technologies is changing how humans can use their 3D printers, as well as the future of automation in the industry.
Big data. Many ‘smart’ 3D printers are also data collection hubs that collect information during the fabrication process and store large amounts of data in the cloud. This data is used by AI to help automate, augment decision making, and improve prints.
Artificial Intelligence. 3D printers can apply AI for numerous benefits in the manufacturing process, such as:
- Optimizing hardware to improve printing speed and quality
- Augmenting human decision making, to determine which parts will drive the most business value and which specific parts are the best candidates to be printed through various additive manufacturing techniques
- Automatically generating tooling jigs or fixtures
- Simulating how parts, before they’re printed, will perform under specific loads
Cloud computing allows organizations to store part files in digital inventories, print to any 3D printer connected to the network, receive software updates automatically, and access their printers’ AI-based features.
Data analytics provide manufacturers with insights into their use of additive manufacturing, such as print times, usage metrics, material consumption, parts uploaded per week, and more.
Additive manufacturing and government programs
Additive Manufacturing Forward. In May of 2022, the Biden administration announced the “Additive Manufacturing Forward'' (AM Forward) initiative in the United States.
The program aims to use today’s advanced additive manufacturing technologies to improve supply chain resilience for American manufacturers.
Seven large American manufacturers — GE Aviation, Raytheon, Siemens Energy, Lockheed Martin, Honeywell, Boeing, and Northrop Grumman — have agreed to accelerate adoption of additive manufacturing (AM) across their small and medium-sized suppliers. To accomplish this the manufacturers will increase their usage of additively manufactured parts, while also lending technical assistance, training, and implementation support.
For more information on how manufacturing organizations are using additive manufacturing to fix their supply chains, read this article.
Further reading
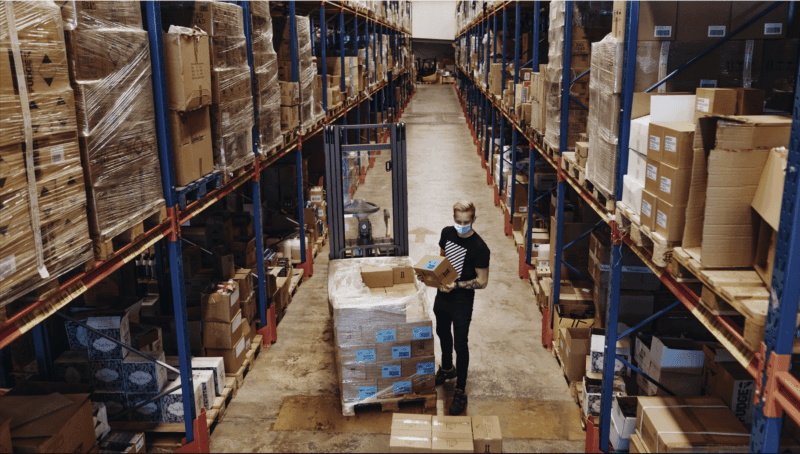
5 Supply Chain Makeovers Powered by 3D Printing
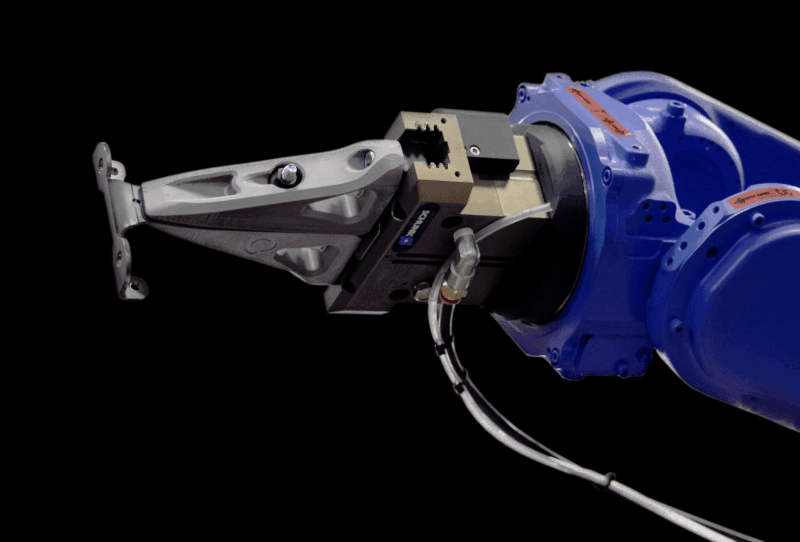
Additive Manufacturing Today: Why Additive, and Why Now?
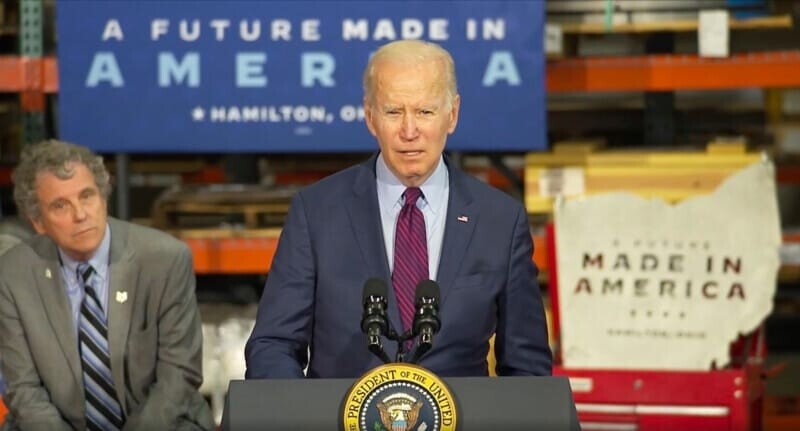
AM Forward Program: Everything You Need To Know
All of the blogs and the information contained within those blogs are copyright by Markforged, Inc. and may not be copied, modified, or adopted in any way without our written permission. Our blogs may contain our service marks or trademarks, as well as of those our affiliates. Your use of our blogs does not constitute any right or license for you to use our service marks or trademarks without our prior permission. Markforged Information provided in our blogs should not be considered professional advice. We are under no obligation to update or revise blogs based on new information, subsequent events, or otherwise.
不错过任何一篇文章
订阅以在您的收件箱中获取新的 Markforged 内容